Reverse Osmosis RO Membrane Particle Fouling
Reverse Osmosis RO Membrane Particle Fouling is caused by organic and inorganic particles contained in the source seawater such as fine debris, plankton, detritus and silt, which cannot pass through the SWRO membranes. All suspended solids which naturally occur in insoluble form, if not removed by pretreatment, would be retained on the feed side of the Seawater Reverse Osmosis RO membrane. Depending on the hydrodynamic conditions on the membrane surface, as well as the size and charge of these particles, they would either migrate along the RO membrane leafs and ultimately exit with the concentrate, or would be trapped on the membrane surface and would begin to accumulate there causing loss of RO membrane productivity over time. This type of foulant can be effectively removed by pre-filtering of the source seawater prior to Seawater Reverse Osmosis RO membrane separation.
Reverse Osmosis RO Membrane Particle Fouling in raw source seawater vary in size. However, most of them, including pico-phytoplankton, are larger than 0.1 μm. Usually, over 90 % of the particulate foulants are larger than 1 μm. A well designed and operating pretreatment system will produce permeate that does not contain particles larger than 20 μm.
Reverse Osmosis RO Membrane Colloidal Fouling
Reverse Osmosis RO Membrane Colloidal fouling is caused by inorganic and organic compounds that naturally exist in suspension and may be concentrated by the SWRO desalination plant separation process, and precipitate on the Reverse Osmosis RO membrane surface thereby causing membrane flux decline over time. Colloidal solids have particle size of 0.001 to 1 μm. For prevention of Reverse Osmosis RO Membrane Colloidal Fouling, SWRO membrane manufacturers usually require RO membrane feed turbidity of less than 0.1 NTU, zeta potential higher than – 30 microvolts (mV) and SDI15 less than 3. The two most common culprits of this type of fouling are colloidal silica and iron.
Typically, seawater collected via open ocean intake does not contain significant amounts iron and silica in colloidal form and this type of fouling usually does not present a challenge in seawater desalination systems with open ocean intakes. Stability of colloids is reduced with the increase in source water salinity, and typical seawater with TDS concentration in a range of 30000 to 45000 mg/L, would contain silica and iron in dissolved and precipitated form rather than in colloidal form. However, if the source seawater is collected via subsurface well intake which is under the influence of brackish coastal aquifer with high content of colloidal silica and/or iron, or it is collected near an area where silt laden river enters into the ocean, than colloidal fouling may become a challenge. Reverse Osmosis RO Membrane Colloidal Fouling could be removed by coagulation, flocculation and filtration, which also applies to Reverse Osmosis RO Membrane particulate fouling.
Reverse Osmosis RO Membrane Mineral Scaling
Reverse Osmosis RO Membrane Mineral Scaling Causes: All minerals contained in the source seawater are concentrated during the process of membrane salt separation. As their concentration increases during the desalination process, ions of Calcium, Magnesium, Barium, Strontium, Sulfate and Carbonate can form sparingly insoluble salts which could precipitate on the membrane surface. The mineral scales that typically form during seawater desalination are these of Calcium Carbonate CaCO3, Calcium and Magnesium Sulfate (CaSO4 and MgSO4), and Barium and Strontium Sulfate (BaSO4 and SrSO4). Formation of mineral scales on the membrane surface is balanced by the high salinity of the seawater which tends to increase the solubility of all salts. This means that the higher the salinity of the source seawater the less likely a mineral scale forms on the membrane surface at typical seawater pH of 7.6 to 8.3 and desalination system recovery of 40% to 50 %. In brackish seawater desalination systems which typically operate at much higher recoveries (75% to 85%), and ionic strength of the source water is relatively lower, mineral scaling is a frequent problem. In typical seawater desalination systems, Reverse Osmosis RO Membrane Mineral Scaling is usually not a challenge, unless source seawater pH would need to be increased to 8.8 or above to enhance Boron removal.
Calcium Carbonate and Magnesium Hydroxide are the most common causes for Reverse Osmosis RO Membrane membrane scaling, when source seawater pH is increased for enhanced Boron removal. Scale formation in this case can be prevented by addition of antiscalant / dispersant to the source seawater. Commonly used parameters which can be used to predict the seawater’s potential to form mineral scaling of calcium carbonate are the Langelier Saturation Index (LSI) and the Stiff and Davis Index (SDI). These indexes are function of the source seawater pH, Calcium concentration, alkalinity, temperature and TDS concentration/ionic strength. It is important to note that although source seawater temperature usually has limited influence on scale formation, when this temperature exceeds 35°C, Calcium Carbonate scale would form at accelerated rate. Metal oxide and hydroxide foulants most frequently encountered during seawater desalination are iron, manganese, copper, zinc and aluminum.
Typically, open ocean seawater contains very low levels of these metal foulants and therefore, if such fouling is encountered on the membrane elements, the usual sources are overdosing of coagulant (iron salt) or corrosion of pipes, fittings, tanks and other metal equipment located upstream of the SWRO system / desalination plant. Iron and manganese fouling may occur if source seawater is collected via subsurface intake from a coastal aquifer which is under the influence of fresh groundwater that contains high levels of these metals in reduced form (iron of more than 2 mg/L (as ferrous) and manganese of more than 0.5 mg/L). This type of scaling problem has been observed in desalination plants with beach well intakes collecting seawater from alluvial coastal aquifers, which are located near the entrance of a river or a creek into the ocean.
If iron and manganese are in reduced form and they are below 1.0 mg/L and 0.1 mg/L respectively, than they can be removed by the SWRO membranes without causing accelerated fouling. However, if iron and manganese are in oxidized form, their levels should be reduced below 0.05 mg/Land 0.02 mg/L, respectively to prevent mineral fouling. Another mineral fouling compound frequently encountered in fresh surface water and brackish aquifers is silica. Open ocean seawater contains silica of less than 20 mg/L and therefore, this compound in not a typical cause of Seawater Reverser Osmosis RO membrane mineral fouling. Silica content above this level usually indicates that the source seawater quality is influenced by a fresh water discharge or coastal aquifer of high silica content located near the desalination plant intake area.
Reverse Osmosis RO Membrane Scaling and Fouling: Causes
Reverse Osmosis RO Membrane Scaling and Fouling have many reasons which will be discussed in this article. First, we need to learn the mechanism of desalination plants. In desalination plants, Seawater is collected from the ocean using either subsurface intakes (wells, intake galleries, etc.) or open ocean intakes. Subsurface intakes naturally pre-screen and pre-filter the collected seawater and thereby they remove coarse debris, and most of the sand and particulates from the seawater. Open ocean intakes which collect ambient seawater directly from the ocean typically have equipment (bar racks, fine traveling screens, micro-screens, and/or strainers) to pre-screen large debris, floating materials, large aquatic organisms, coarse sand, and stringy materials from the source water. As a result, after preliminary screening by the intake facilities seawater typically contains the following five key groups of compounds that could cause Seawater Reverse Osmosis RO membrane scaling and fouling and therefore, would need to be removed by the pretreatment system:
- Particulate foulants (Mainly suspended solids and silt).
- Colloidal foulants: Compounds of relatively small size (0.2 μm to 1.0 μm) that are not in fully dissolved form. When concentrated during the membrane separation process they may coalesce and precipitate on the membrane surface (mainly clay-like substances).
- Mineral scaling foulants: Inorganic compounds (i.e., Ca, Mg, Ba, Sr salts) which during the salt separation process may precipitate and form a scale on the membrane surface (such as Calcium Carbonate CaCO3 and Calcium Sulfate CaSO4, and magnesium hydroxide) or may block the membrane diffusion layer (such as Iron and Manganese).
- Natural organic foulants: Natural organic matter that can attach to and foul the membranes.
- Microbial foulants: Marine organisms and soluble organic compounds that can serve as food to the microorganisms which inhabit in the source water and can form fouling biofilm reducing membrane transport.
Please visit the Hydrodex BLOG to learn more about each key group of compounds and how to prevent your RO membrane from scaling and fouling.
Advantages of Gravity Granular Multi Media Filters in SWRO Pre-treatment
The advantages of gravity granular multi media filters as a pre-treatment in SWRO plants is simply for better removal of algal material from the Seawater. Seawater always contains a measurable amount of algae, which concentration usually increases several times during the summer period and may increase up to 10 times during periods of algal blooms (which may or may not exhibit themselves as red tides).
There is a large variety of algal species in the seawater. Some algal species that occur during red tide events have cells that are relatively easy to break under pressure as low as 0.3 to 0.6 bars. When the algal cells break, they release cytoplasm in the seawater that has a very high content of easily biodegradable polysaccharides. When the amount of polysaccharides released by the broken algal cells exceeds certain level in the filtered seawater, they would typically trigger accelerated biofouling on the seawater reverse osmosis membranes.
Two practical approaches to address this type of problems are: (1) use of dissolved air flotation facility ahead of the pretreatment filters to gently remove algal cells and prevent their breakage (which is preferable); (2) installation of granular activated carbon media layer (cap) on the surface of the filters to remove some of the polysaccharides and other organics in the seawater. Pressure filters usually operate at several times higher pressure than gravity filters. Because the operating pressure of these filters is often higher than the algal cell break pressure threshold, pressure filters would have the disadvantage to cause an accelerated biofouling when filtering seawater of very high algal content. This effect is likely to manifest itself mainly in the summer and during algal blooms when the level of TOC in the source water
exceeds 2 mg/L. Pressure filters are frequently used in Spain and Australia. However, in most successful applications the source water quality is very good (TOC < 1 mg/L, SDI < 4 and turbidity < 4 NTU). Most of the Spanish desalination plant intakes are relatively deep and the algal content in the source water is fairly low. At depth of 10 to 20 m, the concentration of algae is significantly lower than that at the ocean surface – and therefore, as long as desalination plant intake is fairly deep, biofouling caused by breakage and decay of algal biomass may not be as significant problem as it would be for shallow intakes or intakes located at the ocean surface (i.e., near-shore open intakes).
Longer Useful Live of the Filter Structure
Typically, gravity filters are concrete structures that have useful life of 50 to 100 years. Pressure filters are steel structures with a lifespan of 25 years or less. The internal surface of the pressure filters used for seawater desalination is typically lined up with rubber coating that needs to be replaced every 5 to 10 years and inspected occasionally.
Lower Power Use
Because pressure filters typically operate at several times higher feed pressures than gravity filters, the energy use for pressure filtration is proportionally higher.
Higher Solids Retaining Capacity and Better Handling of Turbidity Spikes.
Gravity media filters have approximately two to three times larger volume of filtration media and retention time than pressure filters for the same water production capacity. Therefore, this type of filters can retain proportionally more solids and as a result, pretreatment filter performance is less sensitive to occasional spikes in turbidity of the source seawater. Pressure filters usually do not handle solids and turbidity spikes as well because of their smaller solids retention capacity (i.e., smaller volume of media pores that can store solids before the filter needs to be backwashed). If the source seawater is likely to experience occasional spikes of high turbidity (20 NTU or higher) due to rain events, algal tides, naval traffic, ocean bottom dredging operations in the vicinity of the intake, seasonal change in underwater current direction, or spring upwelling of water from the bottom to the surface, than pressure filters will produce effluent with inferior effluent quality (SDI and turbidity) during such events and therefore, their use would likely result in a more frequent RO cleaning.
Easier to Identify and Fix Filter Operational Problems
Gravity filters are typically covered with light plastic covers that protect the filter cells from direct sunlight.
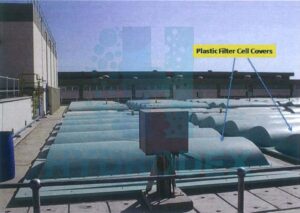
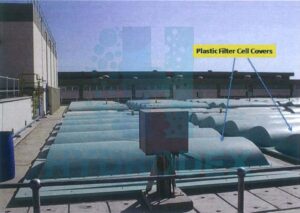
Multi media filter in SWRO covered with a plastic filter cell cover
These covers however, can be easily removed and the filter cells can be inspected visually for irregularities – malfunctioning filter backwash nozzles, weir corrosion, poorly backwashed areas of filter media, formation of “mudballs “, etc. Pressure filters are completely enclosed and very difficult to inspect for the same problems. As a result, these filters have to be designed with higher contingency factor (reserve capacity). A 15 to 20 % reserve capacity is recommended if pressure filters are used to accommodate for potential flow distribution problems and uneven backwash air and water
distribution.
Easier to Accommodate Membrane Pretreatment in the Future
Currently, the existing commercial membrane MF/UF systems have not reached level of development that optimizes their application for seawater pretreatment of difficult to treat source seawater (i.e., seawater exposed to frequent algal blooms). However, in a not so distant future UF and MF membrane technology is expected to evolve into products specifically designed for pretreatment of seawater of high algal content. When this level of maturity of membrane pretreatment technology is reached, it likely would be very advantageous to modify the exiting conventional granular media filters into submersible membrane pretreatment filters. Although currently the market offers both pressure and vacuum-driven pretreatment systems, it is very likely that membrane pretreatment using vacuum-driven membranes would gain wider use, especially for applications where algal blooms in the seawater intake area are frequent events and pressure systems may be less advantageous. As long as gravity filter cells are designed with adequate depth and configuration to accommodate submersible UF/MF membranes, the transition to the new membrane pretreatment technology in the future would require limited additional costs. If pressure pretreatment filters are used, these filters cannot be easily modified into membrane pretreatment systems in the future.
Pressure Granular Media Filters
Pressure filters have filter bed configuration similar to that of gravity filers, except that the filter media is contained in steel pressure vessel. They have found application mainly for small and medium size seawater desalination plants – usually with production capacity of less than 20,000 m3/day. An exception is Spain, where practically all of the pretreatment filters at seawater desalination plants are pressure filters. In most cases for good source seawater quality (SDI < 5 and turbidity less than 5 NTU) the pressure filters are designed as single stage, dual media (anthracite and sand) units. Some plants with relatively poor water quality use two-stage pressure filtration systems. Pressure filters are available in two vessel configurations – vertical and horizontal. Vertical pressure filters (see Figure 15) are customarily used in smaller plants and individual vessels have maximum diameter of 3 m. Horizontal pressure filters (Figure 16) are used more frequently in desalination plants and are more popular for medium and large-size facilities. The largest desalination plant using horizontal pressure granular media filters for seawater pretreatment is the 160,000 m3/day Kwinana SWRO facility in Perth, Australia (see Figure 17). Horizontal filters allow larger filtration area per filter vessel as compared to vertical units. However, usually vertical vessels can de designed with deeper filter media, if deep filters are needed to handle spikes of source seawater turbidity.
Pressure Single-Stage Dual-Media Filters-Key Design Criteria
Compared to gravity media filters, which operate under a maximum water level over the filter bed of up to 2.5 meters, pressure filters typically run at feed pressure equivalent to 15 to 30 meters of water column. The magnitude of the feed pressure is often driven by the suction pressure requirements of the high pressure feed pumps of the downstream SWRO system. One key advantage of the pressure filters is that they could allow avoiding intermittent pumping of the pretreated seawater. A typical SWRO
system with gravity pretreatment filters requires installation of filter effluent transfer pumps to convey the filtrate from the filter effluent well to the high pressure SWRO feed pumps. Use of pressure filters could eliminate the need for such interim filter effluent transfer pumps because the filtrate is already pressure-driven by the intake pumps and the pretreatment filters do not break the hydraulic grade line.
Key design criteria for single-stage dual media pressure filters in small and medium size desalination plants are very similar to these of gravity filters. Design criteria by which pressure filers differ for gravity filters are presented below:
Number of Filter Vessels | 6 to 20 |
Filter Vessel Diameter | 1.2 to 6m (typically 3m) |
Filter Vessel Length 2.5 to 15 m (typically 6 m) | Filter Vessel Length 2.5 to 15 m (typically 6 m) |
Depth of Filter Bed | 0.6 to 0.9m |
Filtration Rate (at Desalination Plant Intake Design Flow) | |
With All Filters in Service | With All Filters in Service |
Head loss Across the Filter Vessel | |
Total Headless Across the Filter | 15 to 30m (20m average) |
Net Headless Available for Filtration | 7.5 to 15m |
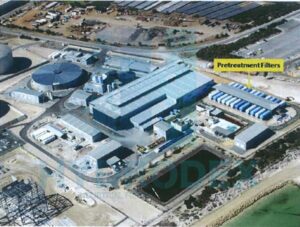
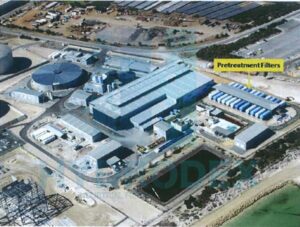
Horizontal Single Stage Granular Media Filter Pressure Vessel Made of Steel in Kwinana SWRO, Australia
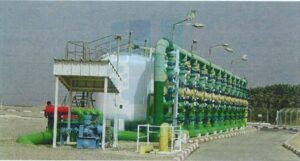
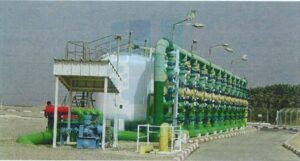
Horizontal Granular Media Filter Pressure Vessel Made of Steel
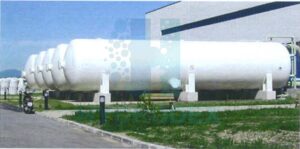
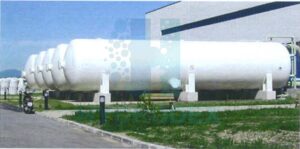
Vertical Granular Media Filter Pressure Vessel Made of Steel
The design criteria presented above are guidelines – media size, depth and configuration, especially for medium and large desalination plants, are recommended to be selected based on pilot testing for the site specific conditions and water quality associated with the project.
- 1
- 2